By Laura E.P. Rocchio
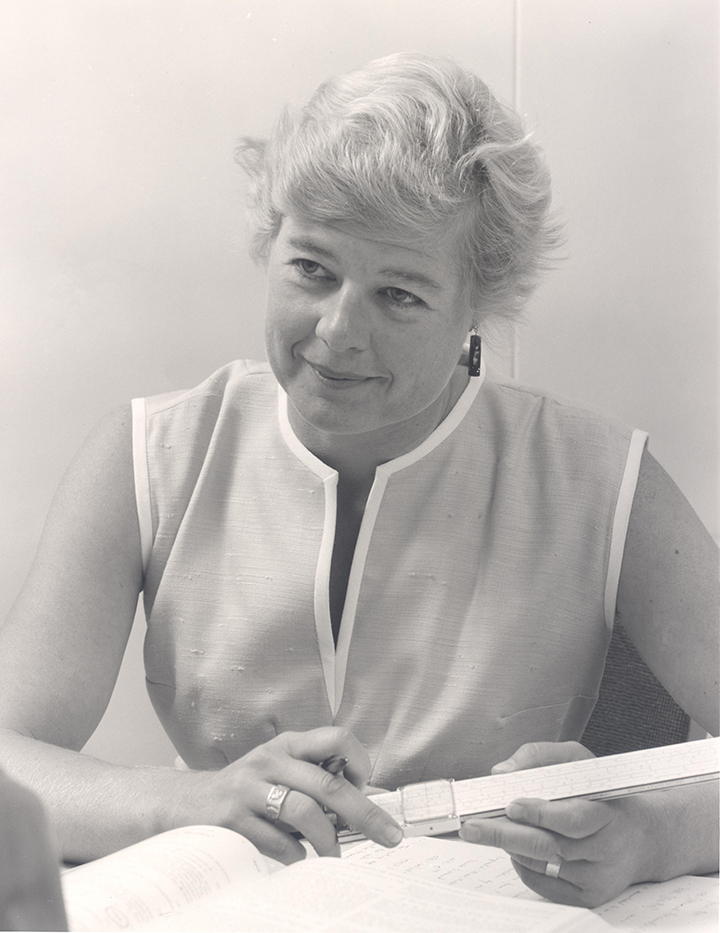
August 07, 2020 • Take out a yardstick. Flip it over to the metric side and measure out 89 centimeters long, 59 centimeters wide and 40 centimeters tall. Imagine an object that size weighing about 105 pounds (48 kg). This should give you a feel for the size of the Multispectral Scanner System that flew on the first Landsat satellite. That’s the compact size of the instrument that initiated repetitive observation of Earth’s landmasses from space—the instrument that has defined much of modern remote sensing.
Now, let’s meet the woman who created it.
At 93, Virginia T. Norwood is an avid birdwatcher, making daily counts of bird species outside of her Topanga Canyon home in Los Angeles. Her six-speed Mazda two-seater is parked nearby; she’s eager to take it out for a drive through the oak-laden hills. She plans to renew her driver’s license—as required every two years at her age—once the Covid-19 pandemic ebbs.
Virginia Norwood has never been one to let challenges stand in her way.
Photons in the Field
The space boom of the 1960s got many companies thinking about aerospace design. At the time, Norwood was part of an advanced design group working for Hughes Aircraft Company in its Space and Communications Division in El Segundo, California. A decade earlier, she had been the first woman to become a member of the company’s technical staff.
The technical staff in her division was given wide latitude to find interesting projects and problems in need of a solution. So, part of Norwood’s job was to know what novel projects NASA was considering. She knew that thanks to the prompting of the Department of the Interior, NASA was interested in acquiring multispectral images of Earth from space for resource management.
It was a problem she was interested in, so she set out to solve it.
After procuring research and development funds, Norwood went about determining exactly what it was that users wanted. Which “colors” or portions of the electromagnetic spectrum did they want to see from space? Did they want to see red, green, and blue portions of the spectrum like human eyes? Did they want infrared or thermal information beyond the scope of human vision?
She embarked on a user-based design study that captured input from the who’s who of resource managers of the era: Ralph Shay from Purdue University; Arch Park from the Department of Agriculture; meteorologist and pollution expert Morris Tepper; and geologist Bill Fischer, hydrologist David Phoenix, and cartographer Alden Colvocoresses, all from the US Geological Survey (USGS).
She spent time in the field with agronomists as they measured light reflectance, counting photons with field spectrometers to assess plant health and uncover the spectral “signatures” of different crop types. She combed the data from laboratories like those at Purdue University and the Environmental Research Institute of Michigan that were flying multispectral imagers on planes.
“I had to talk to people in all kinds of disciplines that I had no idea about before, so I found it utterly fascinating,” she remembers.
She analyzed their input and put together specifications for what spectral bands a space-based instrument should include, what its revisit time should be, and what users needed in terms of geographic pixel location knowledge, spatial resolution, and spectral measurement accuracy.
“I tried to get an intersection of their needs and make the optimal specifications for the scanner itself which would satisfy the broadest range of them,” Norwood explains.
Gathering Earthly Photons from Space
Norwood was convinced that a multispectral scanner offered the most feasible way to meet the instrument specifications that her study had yielded.

NASA scientists and engineers were interested in scanner technology, but skeptical. They had settled on a television-like camera system, known as a Return Beam Vidicon (RBV), as the primary instrument for the first Landsat satellite (known as the Earth Resources Technology Satellite then). This system, built by the Radio Corporation of America (RCA), used traditional camera lenses, shutters, and cathode ray tubes to image a full frame, or scene, at once. Vidicon technology had been proven on the TIROS weather satellites and the Surveyor moon missions. The RBV’s similarity to traditional cameras made it a preferred system for USGS scientists and cartographers as well.
Some forward-thinking engineers at NASA, who were aware of the success of airborne multispectral imagers from midwestern laboratories and familiar with the RBV’s limitations, were willing to take a chance on an experimental scanner payload. So, Norwood and her team at Hughes were awarded a contract to design and build a spaceborne scanner.
Translating an airborne imaging concept to a functional spaceborne scanning system was a monumental task. “This was space—no air, no gravity, significant distances for data signals to travel—it was all new,” Norwood explains.
Norwood’s training, work experience, and position at Hughes made her uniquely qualified to craft the spaceborne scanner.
Norwood was a physicist. In June 1947 she had graduated from the Massachusetts Institute of Technology with a degree in mathematical physics and had taken all graduate courses her last three semesters. Her astute understanding of physics enabled her to find completely new ways to solve problems, as her three US patents attest—the first one for a radar reflector she invented at 22.
She had a solid understanding of the physics of light and myriad trade-offs that a space-based remote sensing instrument must make in terms of spatial, spectral, and radiometric resolutions.
With knowledge garnered from her user interviews, the specifics of the spacecraft’s orbit, and the weight and size allowances for a scanner, she worked the trade-off calculations to figure out what was possible and what could be flight-ready in three years.
“It was heavily dictated by the reflected photons available,” Norwood relates.
On a spacecraft with a total mass of nearly 4000 pounds (1800 kg), the scanner’s mass allocation was meager—just over 100 pounds. The majority of the spacecraft payload space and mass were designated for the primary RBV instrument. Even the data recording system (two gargantuan wideband video tape recorders, weighing in at a combined 152 pounds) had more mass allowance than the scanner.
“We shared space and mass allowance on the satellite with the RCA vidicons and the time we had to get our scanner designed, built, and tested was short. So, the MSS version that first flew was reduced and redesigned to only have four bands,” Norwood recounts.
(A seven-band version of the bigger scanner that Norwood had originally designed would launch a decade later on Landsat 4.)
“It was a matter of putting the biggest telescope you could have, which was 9 inches, and then getting the best spatial resolution within the constraints of the accuracy required, and everyone seemed to want one to three percent accuracy at the lowest light level—which is how I have to think about it when designing the sensor.”
Building the Multispectral Scanner System
Scanners work much differently than cameras. The MSS used a mechanically rocking mirror to scan side-to-side, building up an image line-by-line as the satellite travels forward. The MSS mirror scanned six lines at a time to keep up with the satellite’s orbital speed. Light directed by the scan mirror was sent to a four by six array of optical fibers located at the focal plane; the fibers directed the energy through spectral filters and into light sensitive detectors. In this way, four images, one for each spectral band range, were simultaneously built-up six lines at a time. The energy from photons striking the detectors was sent to a multiplexer that translated the measurements into digital signals, combining the digital data from the 24 detectors into a single bit stream that was relayed to the ground where it was unscrambled back into four images (one for each spectral band).
“It was pretty exotic data handling for 1969,” Ralph Wengler, an early MSS project manager at Hughes shared in a 2005 interview.
To build the scanner she had designed, Norwood capitalized on the engineering expertise at Hughes.
“It was as if I were acquiring pieces of the scanner from various suppliers who all happened to be in different parts of Hughes,” she says. “I recall that my management thought that I was nuts to be going hither and yon for help with pieces of the project; they anticipated difficulties with that approach. But I wanted to get the best expertise available and that ended up working well.”
Hughes had a track-record of building scanners; they had built spin scanners and refined fiber optic technology for the Advanced Technology Satellites (a concept of Verner Suomi’s realized by Hughes engineers). But the ATS weather satellites had a ground resolution of 4000 m and the MSS required precision geolocation at a resolution nearly two orders of magnitude better (79 m). A sizable challenge.
So early on she teamed up with Jack Lansing, a Hughes engineer who had lots of detector experience. Together they refined the MSS design to ensure the best spectral and spatial response possible.
“Jack tucked the different sensors into surprising places so that we could provide the wide spectral response. He was also important in the calibration methods that were critical,” she describes.
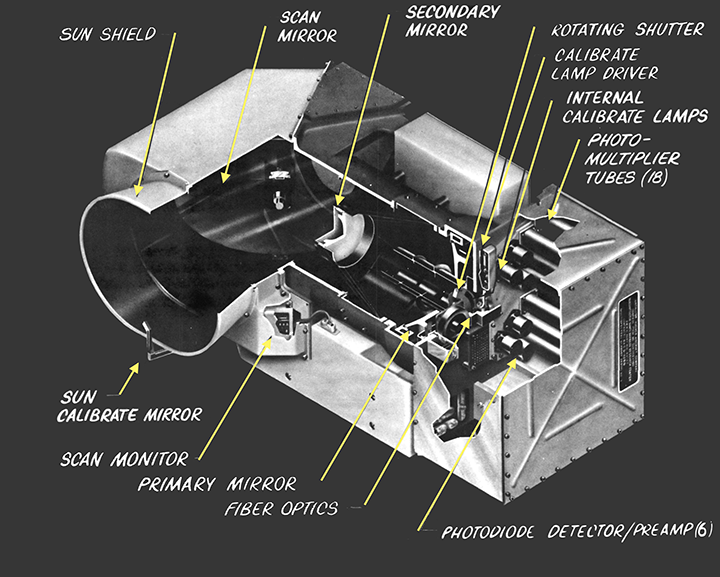
Norwood and Lansing settled on two types of detectors, or sensors. They used silicon photodiodes for the near-infrared band and photomultiplier tubes (PMT) for the green, red, and red “edge” (closer to near-infrared) bands. They would have liked to use all silicon photodiode detectors since the PMTs were heavy, required high voltage, and needed a fair amount of power, but photodiodes couldn’t yet sense visible wavelengths well.
The design of the scan mirror used to direct light from the ground to the detectors was state-of-the-art technology. Norwood had initially envisioned a sinusoidal motion for the mirror, but the finer spatial resolution and spectral accuracy requirements for the MSS made the uneven dwell time of that approach unworkable.
Again Norwood, knew where to go.
“I challenged our ‘house inventor’ S.D. “Webb” Howe to devise a high precision liner scan mechanism,” she says. What he came up with was a “true space mechanism” that drifted by inertia on flex pivots until it banged into bumpers on either side of the scan. It depended on a force-free, low pressure environment so it couldn’t be fully tested in the space testing chamber known as “thermal vac.”
The large beryllium scan mirror was 9 inches by 13 inches and operated at 13.62 Hz, banging back and forth more than 13 times a second. To make such a large mirror light enough to keep within the scanner’s mass allocation, its supporting substrate was hollowed out like an eggcrate.
Later described as “groundbreaking,” the scan mirror met with great consternation at NASA and USGS.
“Just trying to explain how the banging inertial device worked to a vidicon-conditioned generation of electrical engineers was an uphill task,” Norwood wrote in 1991. “We now had derision that had not been heard since the ‘get a horse’ advice in the early days of the automobile.”
Every NASA engineer and USGS scientist that did a site visit to Hughes commented on the noise of the banging mirror, one likening it to a hammer mill.
Norwood assured them that no one could hear it in the vacuum of space.
Norwood’s decision to send the MSS data down digitally was also heavily questioned.
“At that time the very idea of digital encoding met with resistance at NASA,” Norwood recalls.
Norwood decided to quantize the MSS data into 6-bits. Both image data and calibration data (from the onboard internal lamp calibrator) were sent to the ground digitally. Even though the digital MSS data had a small dynamic range (able to show only 64 different radiance values), it could be calibrated, so images could be confidently compared from date-to-date and place-to-place.
The MSS radiometrically-corrected digital data pioneered quantitative satellite remote sensing, taking the science of Earth observation digital, where it has stayed ever since. And calibration methods developed for the MSS have been used as a model for other programs.
But the pre-launch testing for the MSS was more complex than any Norwood had encountered on previous projects.
“Probably the most trying aspect was that of devising tests for verifying radiometric and geometric accuracy at a level that was beyond existing test equipment,” Norwood recalls.
The Path of an Early Female Physicist
Virginia Norwood was born six and a half years after American women had won the right to vote. The country was growing to accept that women could think for themselves.
When she was a young girl, Norwood’s father, an Army officer with a master’s degree in physics, who taught at Carnegie Tech (now Carnegie Mellon University), encouraged her to consider being a physicist or mathematician. He provided her first slide rule at age 9 and many years of math puzzles.
She was good in both math and physics and decided early on that she wanted to go to MIT. Her senior year in high school, she scored very high on her math aptitude tests. A counselor told her she should consider being a librarian. She paid no heed to that advice.
Because MIT was a land grant college, it accepted women at a time when many technical universities did not. Norwood applied and was admitted with a partial scholarship in 1944; three years later she graduated, at age 20, with a degree in mathematical physics.
Finding a technical job in 1947 was a challenge. When asked for her salary requirements at Sikorsky Aircraft in Stratford, Connecticut, she stated the common starting salary for a technician. The interviewer scoffed, telling her no woman at the company had ever made anything close to that. She kept looking.
She ended up selling blouses at a department store in New Haven, until she was hired to teach business arithmetic to returning WWII vets at New Haven Junior College. A highlight of that period was that she was able to audit graduate math classes at Yale where her husband was teaching. “They were very nice to me at Yale, and I benefitted from the opportunity to expand my math foundation,” Norwood relates.
A year after graduating from MIT, both she and her husband were hired by the US Army Signal Corps Laboratories in Fort Monmouth, New Jersey (a federal employer that followed non-discriminatory hiring policies). She took engineering classes through a Rutgers Extension program while living in New Jersey. At the Signal Corps she started in weather radar, and soon designed a radar reflector for weather balloons that discovered previously untrackable winds at 1000 feet. Then she moved into microwave antenna design.
After five years at the Signal Corps Labs, Norwood and her husband headed to California where her husband had grown up. They both worked at Sylvania Electronic Defense Labs in Mountain View. Norwood set up Sylvania’s first antenna lab (buying equipment from a small local outfit run by Bill Hewlet and Dave Packard).
A year later, they moved to Los Angeles. By this time, Norwood’s credentials made her highly sought after. She entertained five or six different employment offers before settling on one from Hughes.
“The offer from Hughes Aircraft Company was not the most money, but I liked them and they were known to have the foremost antenna outfit in the country. They were doing slot arrays and all sorts of exciting, innovative things,” she says.
Her first day on the job there, the parking attendant told her women couldn’t park in the staff lot, that it was for technical staff only. She was a woman, she was the first female member of the company’s technical staff, and from that day forward a woman parked in the lot.
She was at Hughes for 36 years where she designed all kinds of antennae, communication links, optics, the Landsat scanners—and received two more patents. She retired in 1990.
Early on at Hughes when she was made head of the microwave division, a male engineer quit, saying he would not work for a woman. Another engineer there, a Rhodes Scholar no less, told her women, especially ones with children, had no business working at Hughes.
She never let such comments or actions deter her. And soon her creations were on the moon.
“Probably the most exciting time in my career was the day Surveyor landed in 1966—the first fully controlled, soft lunar landing—and started sending back pictures of the moon, on ‘my’ transmitter,” Norwood shares. “I was the engineer in charge of the transmitter, and my group designed the multiplier chain, the amplifier, and the crystal reference for the transmitter that sent the imaging data back to Earth.”
The more Hughes engineers got to know Norwood and the quality of her work, the less they focused on her gender. She was a creative engineer and designer with very sharp scientific and analytic abilities, she could communicate effectively, and she knew how to motivate her team to do their finest, most imaginative work.
As her co-recipient of the 1979 Pecora award, USGS scientist John DeNoyer, described in 2006, “She knew how to handle people. And she was very competent technically too. And you just don’t find people like that every day. Men or women.”
Her advice to aspiring scientists and engineers?
“Really learn the fundamentals of your trade. My solid math and physics foundation served me well for my whole career. Also, enjoy what you’re doing. If you are not having fun and continuing to learn new things, make changes.”
At a time when there were few working moms, Norwood, who had three children, was quite an exception. With instrument testing beyond the scope of computer microprocessors of the time, Norwood and Lansing would have to be at the testing facility all day and night during the expensive MSS thermal vac testing doing performance assessments with calculators, Norwood with her Hewlett Packard 35 calculator, Lansing with his Texas Instrument calculator.
Demonstrating stable MSS performance on the ground proved challenging.
To calm leery customers at NASA and USGS, Norwood and Lansing sent a crew of engineers and technicians out into the field with the engineering model of the MSS, known as the bread board, “to take pretty pictures we could show.”
They mounted the bread board (which looked like “a bunch of boxes”) on a turntable in the back of a truck to simulate the satellite’s progression in orbit and took it all around California scanning vertically to image Lake Tahoe, Yosemite Valley, Half Dome, Glacier Point and San Francisco’s skyline.
“It did confirm that the detectors, the mirror, the data digitizing, and the radiation calibration were all working as designed,” Norwood comments.
The panorama taken of Half Dome hangs on a wall of Norwood’s home to this day.
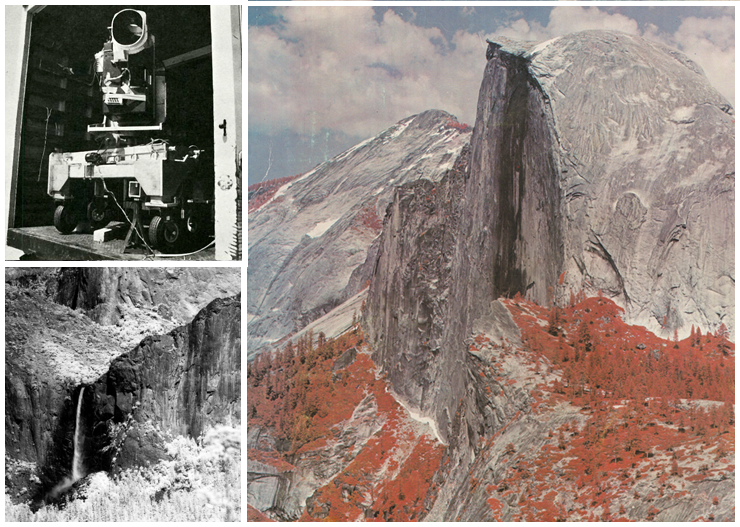
Landsat 1 Launches

On July 23, 1972, Landsat 1 launched with the RBV and MSS aboard. When the first images came down to NASA Goddard Space Flight Center two days later, scientists, engineers, and technicians were amazed by the MSS image quality.
The RBV had been the primary instrument, “But once we looked at the data, the roles switched,” NASA Landsat 1 Project Scientist Stan Freden related in a 2006 interview.
“As we had predicted, when the sensor was launched into the more benign environment of space it evidenced more stable performance both geometrically and radiometrically than we were able to demonstrate on the ground,” Norwood says.
“I never had doubts about the MSS, because I designed it and knew it would work.”
Given the quality of the MSS data, the decision was made not to risk turning the RBV instrument back on after it experienced a near mission-ending electrical anomaly two weeks later.
And so it was that the MSS started the moderate-resolution, visible/near-infrared digital data record of Earth from space that the world has grown accustomed to (along with its refined incarnations).
After a decade of working on Landsat instrumentation, Norwood moved on to other novel projects, but she considers Landsat one of the most satisfying because of its positive societal impacts.
The Landsat Program has been the foremost collaboration of NASA and USGS, and there have been many giants from government, academia, and industry behind its success. For the vision and the political will to implement the Landsat Program, William T. Pecora, former head of the USGS, is accordingly thought of as “The Father of Landsat.”
From a technological standpoint, when you think of the design and engineering that made the Landsat program a success and set the path for modern Earth observation, there is Virginia Norwood.
Is she comfortable with the “Mother of Landsat” moniker?
“Yes. I like it, and it’s apt. I created it; I birthed it; and I fought for it.”
Acknowledgments
We are indebted to Naomi Norwood for spending a full day this July conducting our interview with her mother and providing background details and documents; thanks to the authoring team of the Landsat’s Enduring Legacy book for much of the supporting research including two oral history sessions with Virginia, and to Dr. Darrel Williams for reconnecting our history team with Virginia in 2008.References:
Baumgardner, Marion, Sam Goward, Chris Johannsen, David Landgrebe, and Virginia Norwood. 2009. Landsat Legacy Oral History. West Lafayette, Indiana. Oral History Interview.
Bos, Carrie. 1981. “Norwood: woman of achievement.” Daily News, March 18, 1981.
Goward, Samuel N., Darrel L. Williams, Terry Arvidson, Laura E.P. Rocchio, James R. Irons, Carol A. Russell, and Shaida S. Johnston. 2017. Landsat’s Enduring Legacy: Pioneering Global Land Observations from Space. Bethesda, MD: American Society for Photogrammetry and Remote Sensing.
DeNoyer, John, Len Jaffe, and Bob Porter. 2006. Landsat Legacy Oral History. Greenbelt, MD.
DeNoyer, John, and Virginia Norwood. 2008. Landsat Legacy Oral History. Denver, CO.
Lansing, Jack, and Richard Cline. 1975. “The Four- and Five-Band Multispectral Scanners for Landsat.” Optical Engineering 14 (4):144312.
Markham, B. L., Arvidson, T., Barsi, J. A., Choate, M., Kaita, E., Levy, R., Lubke, M., & Masek, J. G. (2018) Landsat Program. In S. Liang (Ed.), Comprehensive Remote Sensing, vol. 1, pp. 27–90. Oxford: Elsevier.
“NASA – NSSDC – Experiment – Details – Multicolor Spin-Scan Cloudcover Camera (MSSCC)”. NASA. Accessed August 1, 2020.
Norwood, Virginia T. 1974. Multispectral Scanner (MSS) Improved Resolution Study Final Report. Hughes Aircraft Company.
Norwood, Virginia T. 1991. Notes: Early History of the MSS and TM Development.
Phillips, Fletcher, Jack Engel, Ralph Wengler, and Jim Young. 2006. Landsat Legacy Oral History. Santa Barbara, CA.
*5/11/2021 Changed “a version of the bigger seven spectral-band sensor” to “a seven-band version of the bigger sensor” in Gathering Earthly Photons from Space section.